Quasiparticle Spectroscopy from Superfluid Density Measurements
Precision measurements of the London penetration depth in devices provide access to low-energy states inside the superconducting gap, which are the major fingerprints of quantum decoherence.
The Science:
Superconducting materials, like niobium, are key materials for quantum information sciences (QIS) and accelerator technology. Defects and impurities, such as strong affinity for hydrogen and oxygen, are sources for two-level systems (TLS) that are detrimental to quantum coherence. These states manifest themselves as the low-energy bound states inside the superconducting gap in the density of states. This study provides an experimental and theoretical framework showing that these in-gap localized states result in a non-monotonic behavior of the London penetration depth l(T) at low temperatures. Analysis of this non-monotonic behavior is key to detecting and identifying sources of decoherence.
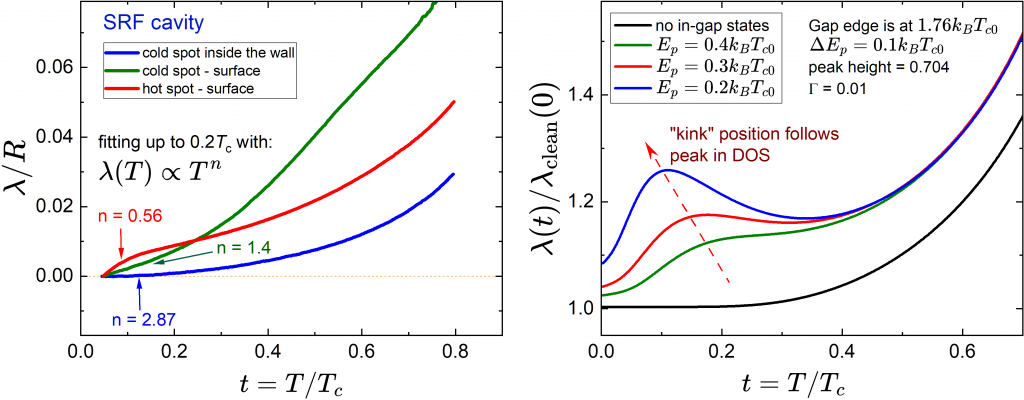
(right) The calculation for three different positions of the in-gap bound states incorporating the TLS peak in the density of states. Deviation from monotonicity is a sign of in-gap states.
The Impact:
This work is the first step in the development of the unique method to characterize the quasiparticle spectra of superconducting qubits. The next step is to introduce microwave perturbation and measure the effect of stimulated non-thermal population of quasiparticles, which enables this technique to be performed under the actual operating conditions of transmons and SRF cavity qubits. If realized, this will enable an efficient non-destructive and scalable characterization of superconducting qubits.
Summary:
Precise measurements of the London penetration depth l(T) on several distinct niobium samples, including single crystals, films, foils and SRF cavity cutouts revealed an unusual non-monotonic behavior at low temperatures. A phenomenological theory that incorporates thermal and bound-state pair-breaking was developed. It is shown that the localized states, modeled as a peak inside the superconducting gap, leads to the observed nonmonotonic behavior. Moreover, it is possible to track the shape and the location of the bound state peak from measured, l(T). Importantly, the peak due to TLS or other bound states can be derived from the microscopic theory developed in the SQMS Center’s James Sauls group. This approach provides a direct test of the microscopic theory, which is crucially important for understanding the mechanisms of quantum decoherence in superconducting devices. In addition, the entire transmon device can be measured non-destructively, thus enabling efficient quality control.
Contact:
Ruslan Prozorov – prozorov@ameslab.gov
Focus Area:
Materials for 2D and 3D Quantum Devices
Institutions:
Ames National Laboratory, Fermi National Accelerator Laboratory, Rigetti Computing
Citation:
“Quasiparticle spectroscopy in technologically-relevant niobium using London penetration depth measurements”, S. Ghimire, K. R. Joshi, A. Datta, A. Goerdt, M. A. Tanatar, D. Schlagel, M. J. Kramer, J. Marshall, C. J. Kopas, J. Y. Mutus, A. Romanenko, A. Grassellino, R. Prozorov, Materials for Quantum Technology 4, 045201 (2024)
https://doi.org/10.1088/2633-4356/ad8c0c
Acknowledgement:
This work was supported by the U.S. Department of Energy, Office of Science, National Quantum Information Science Research Centers, Superconducting Quantum Materials and Systems Center (SQMS) under Contract Number DE-AC02-07CH11359. M A T and K R J were supported by the U.S. Department of Energy (DOE), Office of Science, Basic Energy Sciences, Materials Science and Engineering Division. The research was carried out at the Ames National Laboratory, operated for the U.S. DOE by Iowa State University under Contract # DE-AC02-07CH11358.