Materials for 2D and 3D quantum devices
Our goals: Understand and mitigate the key limiting mechanism of coherence in superconducting qubits, such as losses in two-level systems in oxides, non-thermal quasiparticles, and the bulk substrate.
- Demonstrate a jump of at least an order of magnitude in coherence times with superconducting 2D transmons with coherence up to miliseconds, along with a decrease in performance spread to enable the high coherence multiqubit processors.
- Demonstrate SRF cavities with coherence times up to tens of seconds.
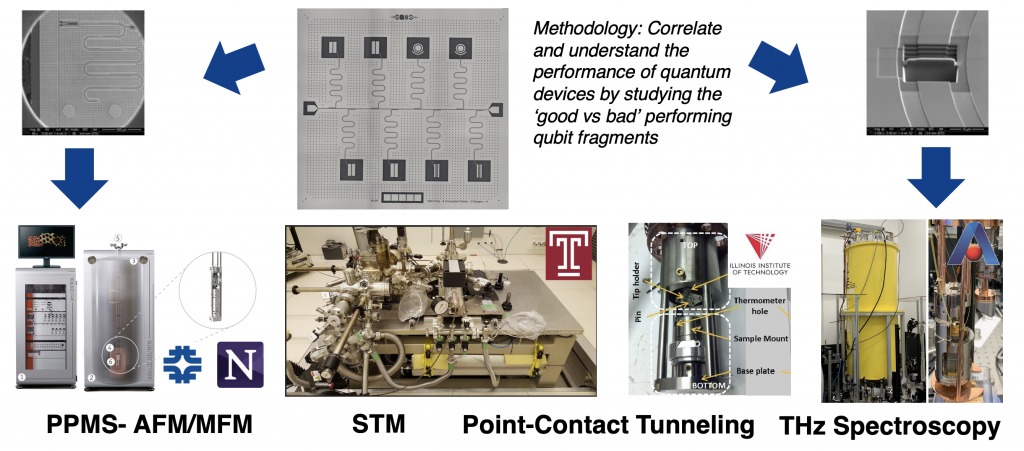
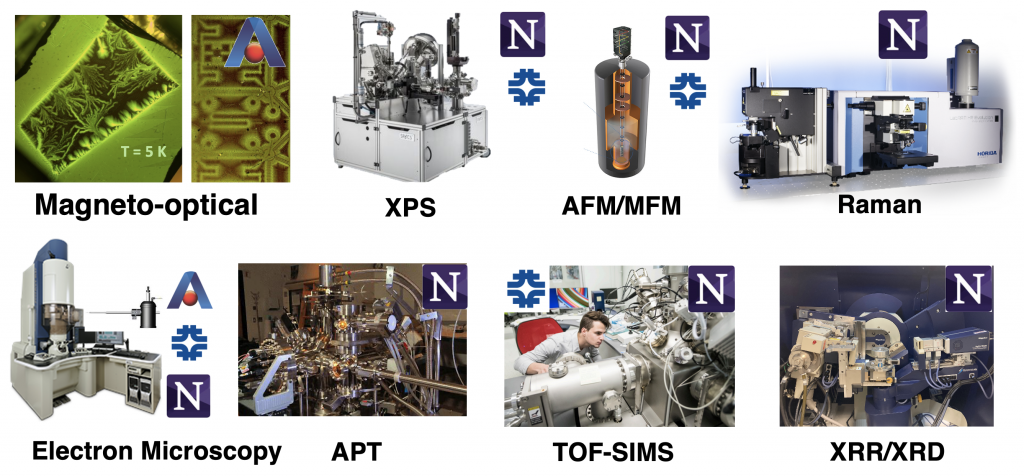
The SQMS collaboration has launched the largest systematic investigation into the origin of decoherence of materials. By studying performance differences of state-of-the-art qubits with the world’s most advanced materials and superconducting characterization techniques, together with modeling efforts, the Center is building a hierarchy of loss mechanisms that informs how to fabricate the next generation of high-coherence qubits and processors.
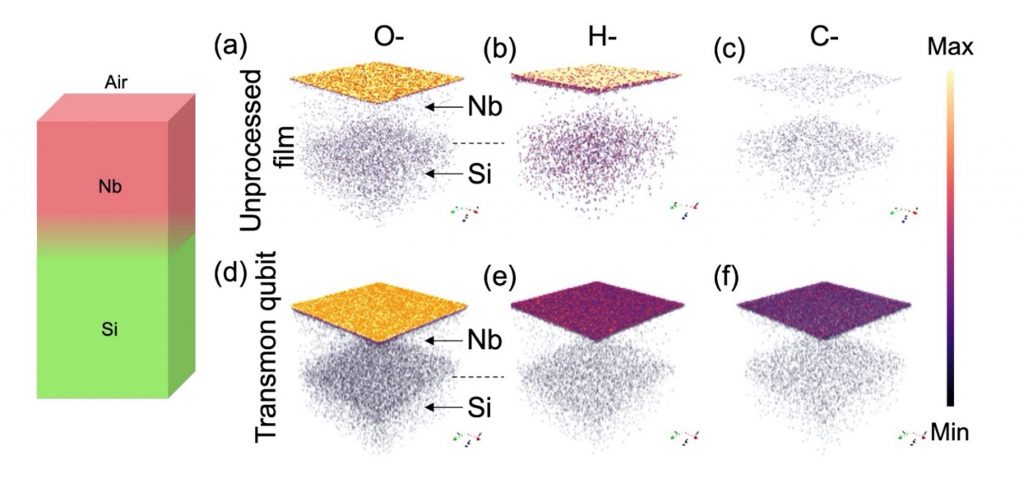
As an example, SQMS scientists used time-of-flight secondary ion mass spectrometry, ToF-SIMS, for the first time to reveal impurities such as oxygen, hydrogen, carbon, chlorine, fluorine, sodium, magnesium, and calcium by building a three-dimensional reconstruction of the qubit at the atomic level. This technique involves bombarding ions at a qubit to chip away at it. The resultant ions ejected from the qubit are detected with part-per-million sensitivities. As another example, by employing techniques such as electron microscopy, atomic force microscopy, and x-ray diffraction for the first time at cryogenic temperatures to analyze qubits, we have identified new features such as nano-hydride formation as one of the factors that reduce coherence times. Various other advanced characterization techniques applied to state of the art transmon qubits have shed light on several important sources of decoherence in oxides, interfaces and substrates.
The Nanofabrication Taskforce
The advances in the understanding of sources of decoherence from the materials’ efforts guide the development of new processes for quantum device fabrication. SQMS has established the first national Nanofabrication Taskforce, bringing together qubit fabrication experts from Fermilab, NIST, Northwestern, and Rigetti Computing. This taskforce focuses on making devices with new standard processes and verifying the reproducibility of results across four different state-of-the-art foundries. Results include newly developed innovative fabrication techniques, such as niobium surface encapsulation and silicon surface passivation, which have systematically improved the performance of transmon qubits to world-leading values.
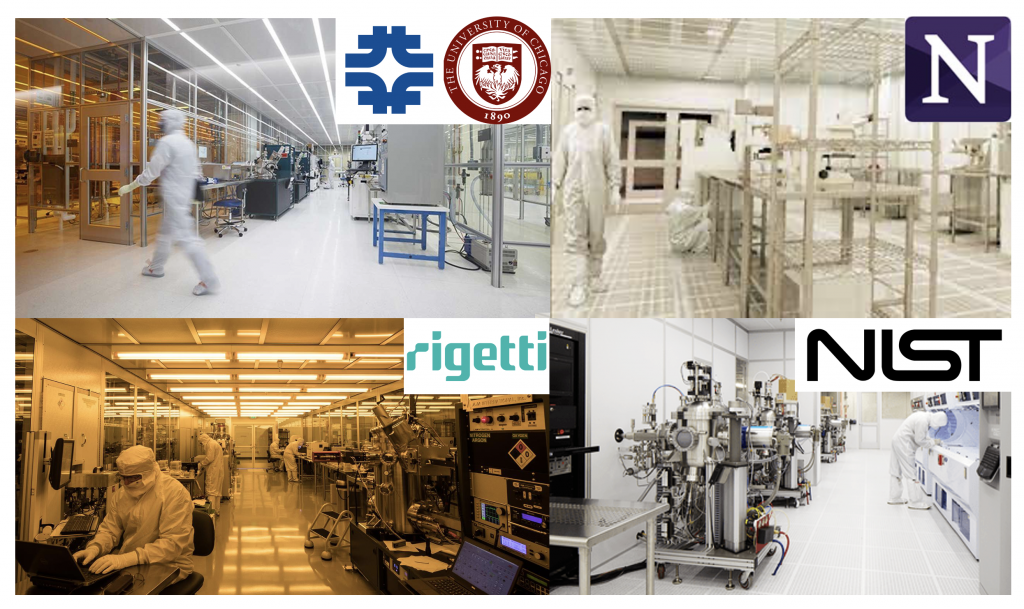
The Round Robin Experiment
SQMS has launched the Round Robin experiment: the first international qubit chips exchange to study and compare the performance of identical qubits at different test sites worldwide. The collaboration has worked to streamline and standardize test setups, control systems, qubit fixtures, packaging and measurement protocols. The underground INFN facilities allow for comparison to above-ground for possible effects of environmental radiation, including possible radioactivity coming from the chip package and fridge environment. Combining measurements with advanced diagnostics, we are shedding new light on both intrinsic and extrinsic origins of decoherence and performance variations.
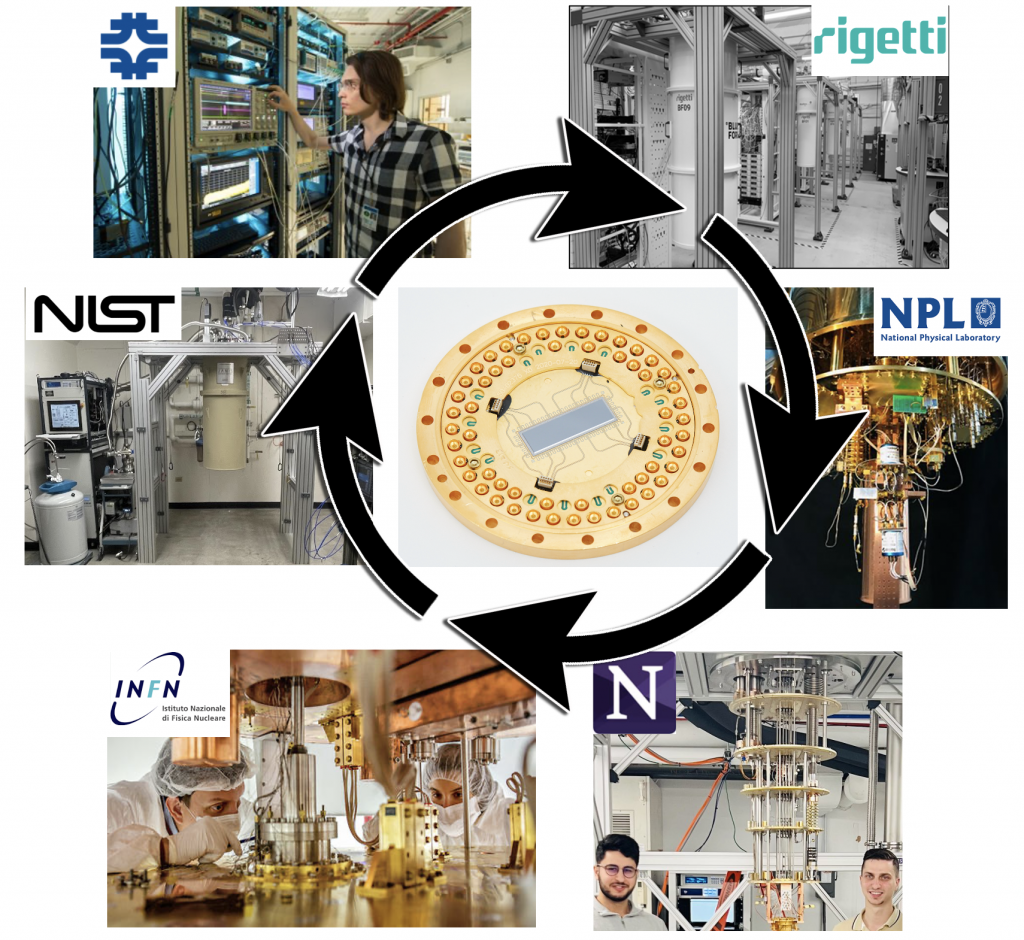