Quantum for fundamental scientific discovery
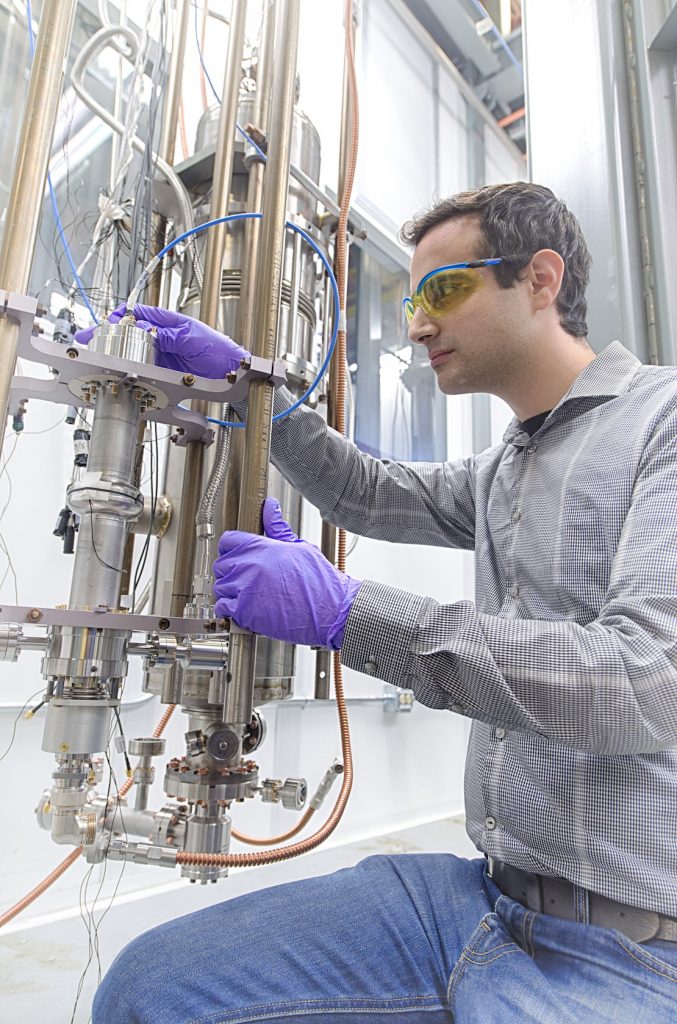
Our goals: Explore quantum technology advancements for fundamental physics.
- Are there new interactions and particles?
- What makes up the invisible Dark Matter in our Universe?
- What are the limits of quantum entanglement and the emergent properties of complex entangled states?
- Can we detect high-frequency gravitational waves?
Use the science drivers to push the development of sophisticated quantum devices and control techniques.
Demonstrate quantum advantage for fundamental science problems by:
- Pushing the exclusion boundary for axion or dark photon searches by more than one order of magnitude from the current state of the art;
- Push beyond the limits of quantum entanglement, building record-size cat states to explore the quantum-classical boundary.
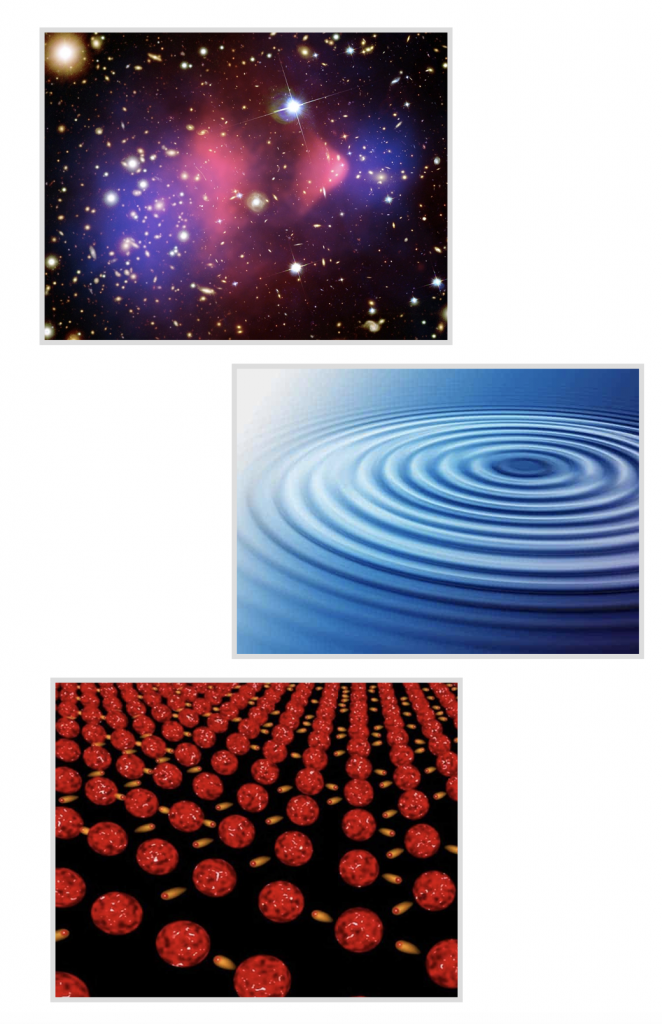
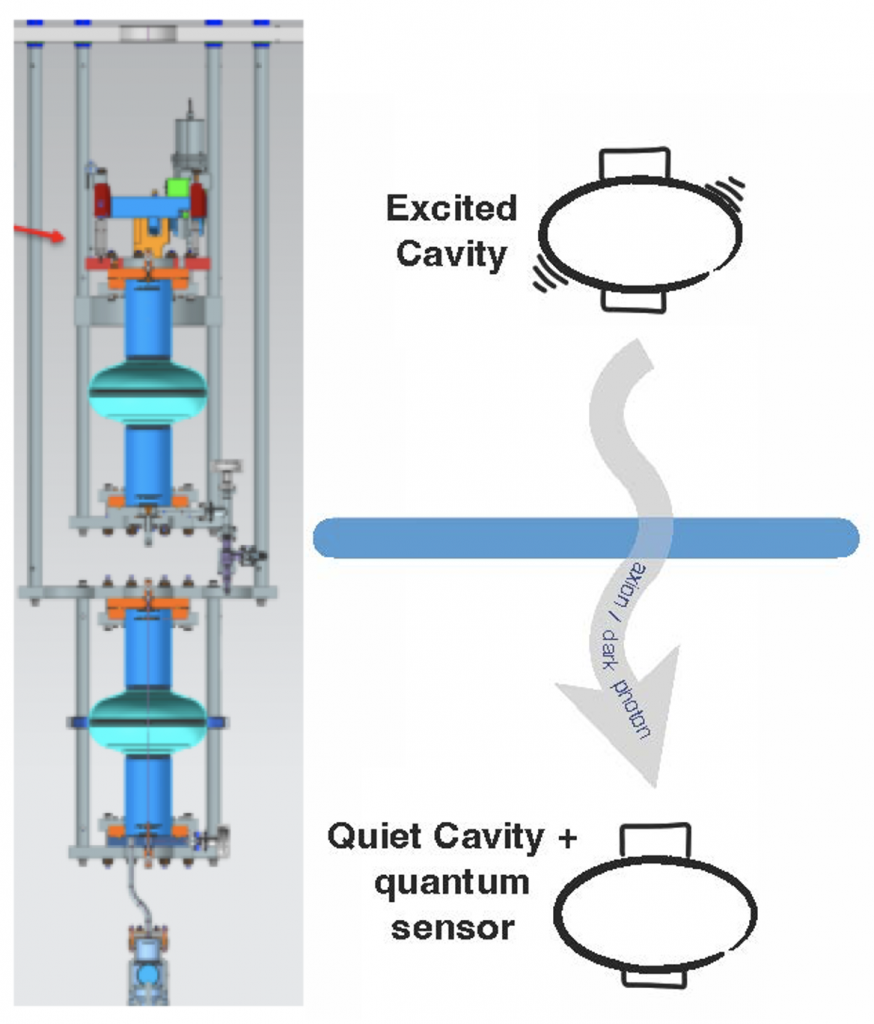
Dark matter and dark photons
According to Newton’s law of gravity, the velocity that a planet needs to maintain to remain in a stable orbit depends on the total mass that resides in the center of its orbital motion. In 1930, Fritz Zwicky made the remarkable observation that galaxies in the Coma cluster orbit much faster than their combined mass can explain. Zwicky postulated the existence of a new form of invisible matter, which he named Dark Matter.
This strange form of matter only interacts via the force of gravity, and so does not produce any visible or other types of radiation to be detectable. Support for the existence of Dark Matter comes from astronomical observations that clusters of galaxies bend light more than expected. Dark Matter was abundantly produced during the Big Bang. As Dark Matter cooled, it began gravitational clumping, so galaxies started to form. Thus, Dark Matter is essential for a galaxy’s existence.
Dark Matter constitutes 23% of all the matter and energy in nature, as compared to 5% of ordinary, familiar matter. But its constituents are completely unknown and undetected, remaining one of the dominant mysteries of science today.
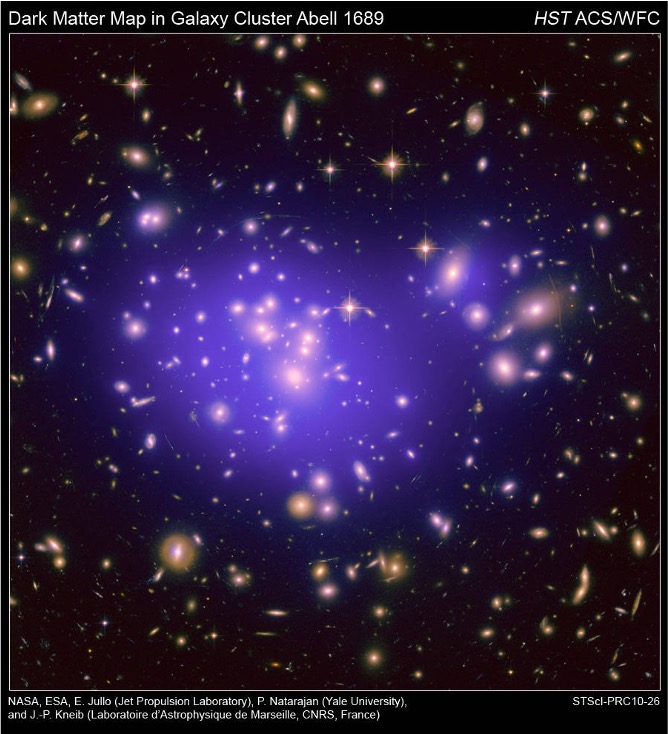
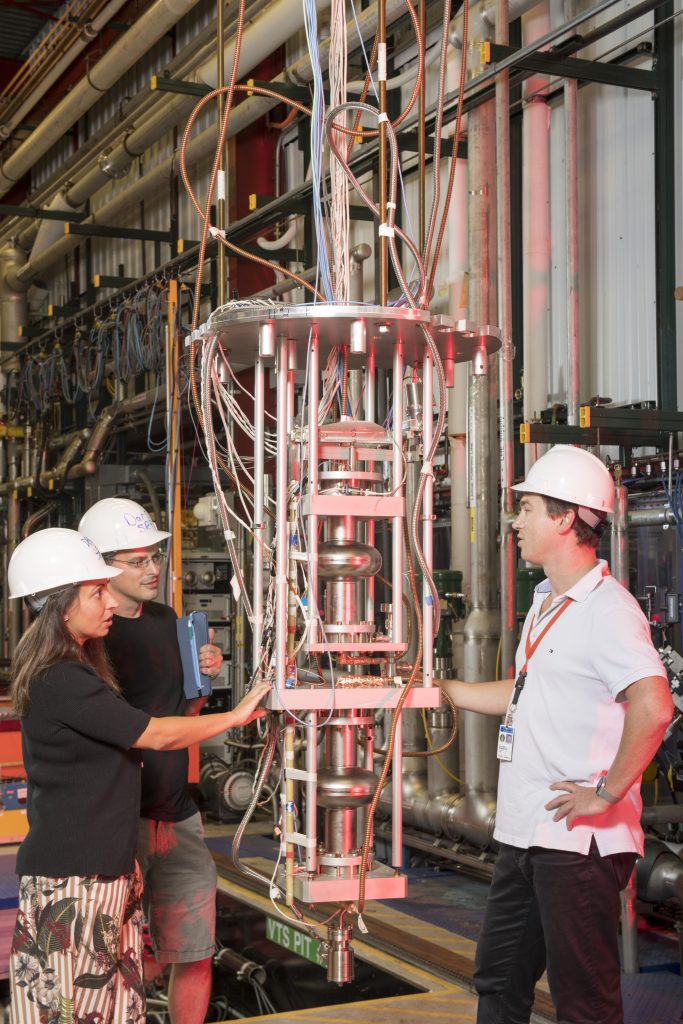
Dark photons?
There are various theoretical candidates for dark matter particles under exploration with special experiments. One candidate is the dark photon. Its fascinating property (if it exists) is that it can go through walls! It is the invisible counterpart to photons. In the Dark SRF experiment, SQMS researchers are using a pair of superconducting resonators, hunting for signals of dark photons, and have already established world-record sensitivity in a certain mass range. With qubit sensors, these experiments are further increasing the sensitivity of detection, leading to discovery potential.
SQMS building devices for ultimate quantum sensing
Another candidate for Dark Matter is the Axion, named after a laundry detergent, to clean up a key problem in high-energy physics! It is a hypothetical particle with a mass 100 billion times less than that of the electron.
Axions were postulated to explain why Charge-Parity (CP) violation is not observed in strong interactions, although it should be – according to the Standard Model. It also plays a role in cosmology. Even though their mass could be in the range of micro-eV if axions exist, there could be so many of them in the Universe, that they contribute a large proportion of the overall mass of dark matter in the universe, and therefore 23% of all matter. By saturating a region with a very strong electromagnetic field, the axion may decay into two photons, which could be detected by quantum sensors that have the capability to detect single photons.
Discovery of axions would re-write the laws of particle physics and cosmology.
A strong superconducting magnet will coax dark matter out of hiding and convert axions into light particles inside a superconducting microwave quantum resonator. Equipped with ultrasensitive, low-noise quantum electronics, a detector can be tuned to different frequencies corresponding to axions of different masses. The expected signal is at 10-23 watts or less.
SQMS is pushing several experiments in collaboration with INFN and other institutions, with cavities and qubits, some of which have already demonstrated world-leading devices and sensitivity and discovery potential for dark sector particles and axion-like particles, and even gravitational waves detection.
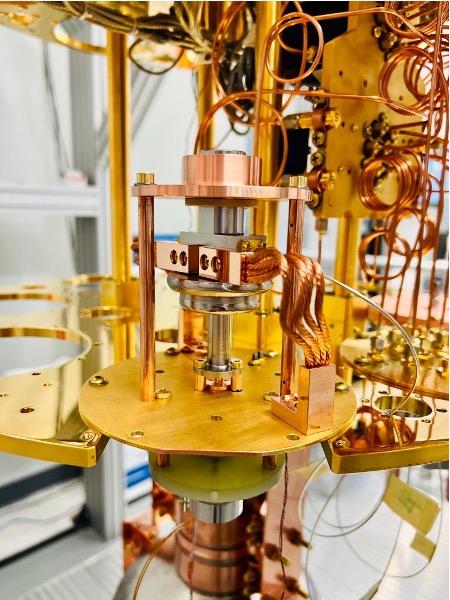